Gene circuit engineering is a captivating frontier in synthetic biology, offering the potential to program living cells much like we program computers. By constructing synthetic networks of genes—akin to electronic circuits—scientists can dictate cellular behaviors, opening up a realm of possibilities in research, medicine, and biotechnology. Let’s embark on an in-depth exploration of this fascinating field, delving into its foundational principles, diverse applications, and the ethical considerations that accompany its rapid advancement.
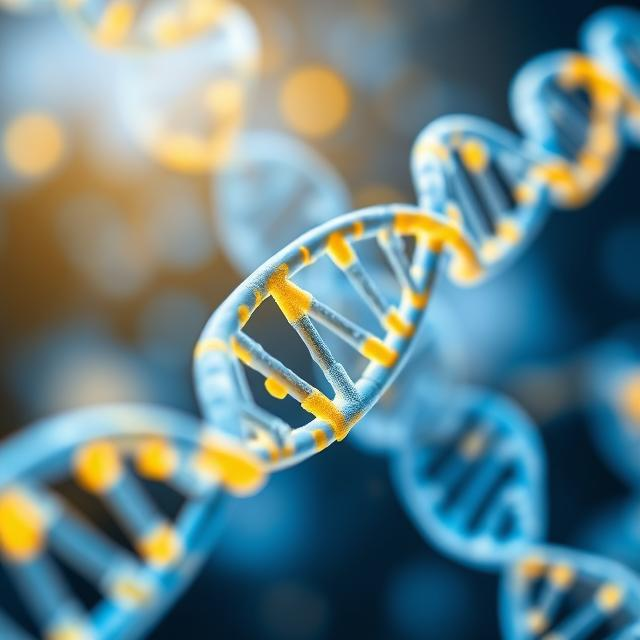
Foundations of Gene Circuit Engineering
At its essence, gene circuit engineering involves designing and assembling networks of genetic components that can process inputs (such as environmental signals) and produce specific outputs (like the production of a protein). These synthetic circuits emulate the logical operations of electronic circuits, utilizing elements such as promoters (which initiate gene transcription), repressors (which inhibit gene expression), and feedback loops (which regulate the circuit’s activity) to achieve desired cellular functions.
The design process typically encompasses several critical steps:
- Identification of Biological Parts: Selecting genetic elements that serve specific functions, such as DNA sequences coding for proteins or RNA molecules. This selection is akin to choosing the right components for an electronic circuit.
- Modeling and Simulation: Employing computational tools to predict how the proposed circuit will behave within the complex environment of a living cell. This step is crucial for anticipating potential issues and refining the design before actual implementation.
- Assembly and Testing: Synthesizing the genetic components and introducing them into host cells to observe functionality. This phase involves iterative testing and modification to ensure the circuit performs as intended.
Advancements in DNA synthesis and computational biology have significantly streamlined this process, enabling the creation of more complex and reliable gene circuits. For instance, the development of design automation tools has facilitated the rapid prototyping of genetic circuits, reducing the time and effort required to bring new designs to fruition.
Applications in Biomedical Research
Gene circuits have become invaluable tools in biomedical research, providing deeper insights into cellular processes and disease mechanisms. A notable example is the work conducted at the Neural Circuit Engineering Lab at Drexel University, where researchers utilize gene circuits in Drosophila melanogaster (the common fruit fly) to study synapse formation in the central nervous system. Due to the conservation of these mechanisms across species, findings from fruit fly models can shed light on human neurological disorders and neurodegenerative diseases.
By manipulating gene expression in specific neural circuits, scientists can observe the resulting behavioral changes, thereby elucidating the roles of particular genes in neural development and function. This approach has provided significant insights into conditions such as Alzheimer’s disease and schizophrenia, offering potential pathways for therapeutic intervention.
Therapeutic Innovations
The therapeutic potential of gene circuit engineering is vast, particularly in developing targeted cancer treatments. Companies like Senti Biosciences are at the forefront of this endeavor, creating customizable gene circuits capable of distinguishing between healthy and diseased cells. Their “logic gating” technology enables therapies to recognize multiple cancer targets and activate or deactivate accordingly, minimizing damage to healthy cells. For example, Senti Bio’s lead candidate, SENTI-202, is an allogeneic CAR-NK cell therapy designed to target acute myeloid leukemia (AML) by recognizing specific cancer markers.
Another groundbreaking application involves the development of synthetic gene circuits that can detect and respond to specific oncogenic signals, such as those from mutated RAS proteins commonly found in various cancers. Researchers have designed circuits that, upon sensing aberrant RAS activity, trigger the expression of therapeutic proteins specifically within cancer cells, thereby reducing collateral damage to healthy tissues. This precision medicine approach holds significant promise for improving cancer treatment outcomes.
Advancements in Gene Circuit Design
Recent progress in gene circuit design has focused on enhancing precision, reliability, and scalability. One notable advancement is the development of Synthetic Translational Coupling Elements (SynTCE), which significantly improve the precision and integration density of genetic circuits. By enabling more accurate control of gene expression, SynTCE facilitates the construction of complex synthetic networks with predictable behaviors.
Additionally, the engineering of inducible gene expression systems has been pivotal. These systems allow external control over gene expression through small molecules, providing temporal regulation essential for applications requiring precise timing, such as in gene therapy and biotechnology manufacturing. For instance, a high-performance inducible gene expression system can be activated or deactivated in response to specific chemical signals, ensuring that therapeutic genes are expressed only when needed.
Furthermore, the integration of machine learning algorithms into gene circuit design has revolutionized the field. By analyzing vast datasets, machine learning models can predict the behavior of genetic circuits, identify potential failure modes, and suggest optimizations, thereby accelerating the design process and enhancing the robustness of synthetic biological systems.
Educational Initiatives
As gene circuit engineering continues to evolve, educational programs are emerging to equip the next generation of scientists with the necessary skills. Courses like Engineering Genetic Circuits: Design offered by the University of Colorado Boulder provide students with a comprehensive understanding of the principles and practices involved in constructing genetic circuits. These programs cover topics ranging from the basics of cellular biology to the intricacies of assembling complex genetic devices, fostering a new cadre of bioengineers capable of advancing the field.
Ethical Considerations
With great power comes great responsibility, and the advancement of gene circuit engineering brings forth important ethical considerations. Public perception and oversight are paramount, as the ability to modify living organisms at the genetic level raises concerns about safety, consent, and potential misuse. Transparent communication and robust regulatory frameworks are essential to address these concerns and build public trust.
Additionally, the potential for dual-use—where technologies intended for beneficial purposes could be misapplied—necessitates vigilant monitoring and control. The scientific community must proactively engage in ethical discourse, ensuring that the development and application of gene circuit technologies align with societal values and do not inadvertently cause harm.