Escherichia coli remains one of the most studied organisms in biotechnology. Recent developments showcase its versatile application in producing DNA vaccines, optimizing enzyme fermentation processes, and even enabling novel CRISPR enzyme production. This post explores the latest breakthroughs in E. coli-based technologies—from DNA vaccine trials and transcutaneous immunization strategies to leveraging bacterial sensors for CRISPR diagnostics. We’ll break down the science, highlight key process innovations, and discuss the potential clinical and industrial impact of these advances.
E. coli DNA Vaccines: From Clinical Trials to Market Challenges
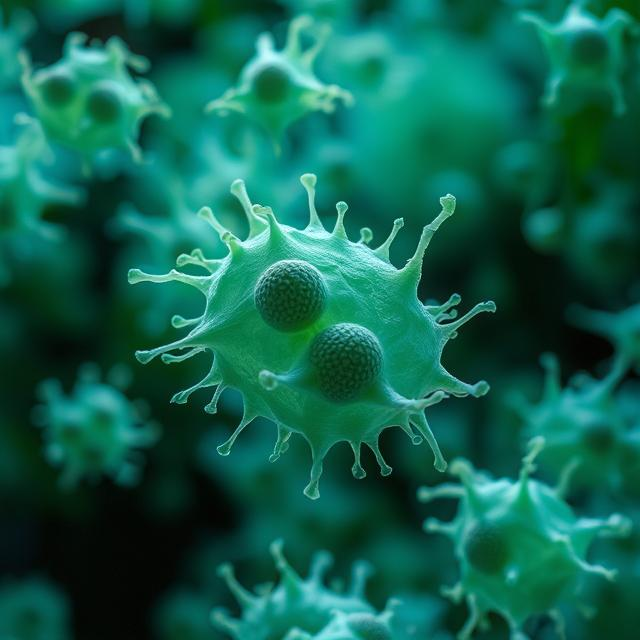
E. coli has emerged as a promising platform for DNA vaccine production. Leveraging its rapid growth and well-understood genetics, researchers and companies have attempted to develop vaccines that can be produced efficiently and at scale. A recent update on an extraintestinal pathogenic E. coli vaccine Phase 3 clinical study underscores both the potential and challenges of this approach.
Key Points:
- Phase 3 Update and Forward-Looking Statements:
- Sanofi’s press release emphasized forward-looking statements about the vaccine’s development and future potential.
- The communication detailed expectations regarding clinical outcomes, product development plans, and market performance.
- Investors were cautioned about inherent risks such as regulatory uncertainties, variable clinical results, and market dynamics.
- Trial Termination and Financial Impact:
- In a significant setback, Sanofi, in partnership with J&J, abandoned a trial of an E. coli vaccine after disappointing data emerged.
- The financial implications were substantial: Sanofi had invested heavily—$175 million upfront plus milestone payments—and later recorded a $250 million charge due to the trial’s termination.
Clinical Implications:
While the termination of the trial highlights the challenges in developing effective DNA vaccines using E. coli platforms, the research continues to provide invaluable insights. Understanding why the trial failed could lead to improved formulations and strategies in the future, ultimately contributing to more robust vaccine candidates.
E. coli Enzyme Fermentation: Uncovering New Cellular Phenomena
Beyond vaccine development, E. coli is instrumental in enzyme fermentation processes that can impact both industrial applications and fundamental biological research. A fascinating study published in Nature demonstrated how electrolysis products from a platinum electrode can inhibit cell division in E. coli.
Research Highlights:
- Electrolysis and Transition Metal Compounds:
- The study identified that certain group VIIIb transition metal compounds, when present at concentrations as low as 1–10 parts per million, significantly inhibit cell division.
- This inhibition leads to the formation of long, filamentous cells—up to 300 times the normal length—without markedly affecting overall growth.
Implications for Fermentation Processes:
- Bioprocess Optimization:
- Insights from these findings can help optimize fermentation conditions where control over cell division and morphology is critical.
- By fine-tuning metal ion concentrations and electric field parameters, it may be possible to manipulate bacterial physiology to enhance the production of desired metabolites or recombinant proteins.
- Fundamental Biology:
- The discovery opens new avenues for studying the impact of electrical stimuli and metal compounds on bacterial cell division.
- This research not only deepens our understanding of bacterial stress responses but also offers potential applications in industrial biotechnology.
E. coli Fermentation for DNA Vaccine Antigens
E. coli continues to be a workhorse for the production of recombinant proteins, including vaccine antigens. Many research institutions and biotech companies have optimized expression platforms that utilize E. coli to produce high yields of recombinant proteins for DNA vaccines.
Process Overview:
- Expression Platforms:
- The Antigen Discovery Unit at Baylor College of Medicine (BCM) is a prime example of how E. coli, along with yeast and mammalian cell systems, is employed to optimize protein expression.
- Researchers initially develop small-scale seed stocks by assessing yield and protein solubility, which then inform large-scale production strategies.
- Scale-Up and Purification:
- Once optimized, these seed stocks are transferred to the Process Development Unit for large-scale antigen production.
- This scalable production is critical for meeting the demands of pre-clinical trials and potential commercial vaccine manufacturing.
Advantages of the E. coli Platform:
- Cost Efficiency:
- Rapid growth and ease of genetic manipulation make E. coli a cost-effective system for protein production.
- High Yield:
- Optimized fermentation conditions allow for high yields of recombinant proteins, which is crucial for vaccine development.
- Process Flexibility:
- The ability to quickly scale from small pilot batches to industrial production batches ensures that promising vaccine candidates can be rapidly developed and tested.
Transcutaneous Immunization: A Needle-Free Vaccine Delivery Strategy
An innovative approach to vaccine delivery is transcutaneous immunization (TCI), which offers a needle-free method to stimulate robust immune responses. Recent studies have explored the use of a patch containing heat-labile enterotoxin (LT) derived from E. coli for this purpose.
How Transcutaneous Immunization Works:
- Mechanism:
- The vaccine patch is applied to the skin, an area rich in immune cells.
- The LT antigen, when delivered transcutaneously, stimulates both systemic and mucosal antibody responses.
- Advantages of TCI:
- Needle-Free Delivery: Reduces the risks and discomfort associated with traditional injections.
- Enhanced Immune Response: Targeting the skin’s immunologically active environment can lead to more robust and rapid antibody production.
- Simplified Administration: Patches can be easily administered, potentially increasing vaccine uptake in mass immunization campaigns.
Clinical Potential:
- Human Applications:
- Preliminary studies have demonstrated safe and effective use of TCI in humans, resulting in significant LT-antibody responses.
- Broad Applicability:
- This approach could be adapted for various vaccine antigens, potentially transforming how vaccines are delivered and administered.
E. coli-Based CRISPR Enzyme Production: Harnessing Bacterial Sensors
E. coli’s natural abilities extend into the realm of CRISPR enzyme production. Recent developments have focused on using E. coli to produce CRISPR/Cas enzymes more efficiently while also capturing valuable gene expression data.
The Record-seq Technology:
- Concept:
- As explained by Randall Platt, the Record-seq technology leverages E. coli’s extensive sensor systems to infer human environmental conditions from bacterial gene expression.
- By analyzing the transcriptome of E. coli under different conditions, researchers can detect specific stress responses or environmental cues, such as those induced by colitis or dietary changes.
Key Features:
- Bacterial Sensors:
- E. coli possesses a wide array of sensors capable of detecting minute changes in the environment.
- These sensors regulate gene expression, allowing the bacteria to adapt rapidly to external stressors.
- Applications in CRISPR Production:
- Harnessing these natural sensors can improve the production and optimization of CRISPR/Cas enzymes.
- By monitoring gene expression profiles, researchers can fine-tune fermentation conditions to maximize enzyme yield and activity.
- Predictive Capabilities:
- Record-seq enables the prediction of environmental conditions based on bacterial responses, potentially informing the design of more robust CRISPR-based diagnostic tools and therapeutic systems.
Industrial and Research Implications:
- Streamlined CRISPR Manufacturing:
- E. coli-based production systems could simplify the manufacturing of CRISPR enzymes, reducing both time and cost.
- Enhanced Diagnostic Tools:
- The ability to capture and interpret bacterial gene expression can lead to the development of “living diagnostics” that monitor human health via microbial indicators.
- Broader Biotechnological Applications:
- Insights gained from Record-seq and similar technologies could be applied to other bacterial systems, further broadening the impact of CRISPR-based technologies.
Process Flow: From Fermentation to Product Delivery
A typical workflow for E. coli-based vaccine antigen and CRISPR enzyme production involves several key stages. Here’s an overview:
- Small-Scale Expression and Screening:
- Seed Stock Generation:
- Small batches of E. coli are cultured to assess protein yield and solubility.
- Initial Screening:
- Researchers select the most promising candidates for further development.
- Seed Stock Generation:
- Scale-Up Production:
- Process Development Unit (PDU):
- Selected seed stocks are transferred to a PDU for large-scale fermentation.
- Optimized Fermentation:
- Fermentation conditions are fine-tuned to maximize protein production and enzyme activity.
- Process Development Unit (PDU):
- Purification and Quality Control:
- Recombinant Protein Purification:
- Proteins are purified using established biochemical techniques to ensure high purity.
- Quality Assessment:
- Purified proteins undergo rigorous quality control to confirm efficacy and safety.
- Recombinant Protein Purification:
- Clinical or Industrial Application:
- Vaccine Antigens:
- Purified vaccine antigens are incorporated into formulations for pre-clinical and clinical trials.
- CRISPR Enzymes:
- Optimized CRISPR/Cas enzymes are utilized in gene editing applications or further processed into diagnostic tools.
- Vaccine Antigens:
Benefits and Challenges in E. coli-Based Bioprocessing
E. coli’s extensive use in biotechnology is well founded, yet several challenges remain:
Benefits:
- Cost-Effective Production:
- The rapid growth and ease of genetic manipulation in E. coli make it a cost-efficient platform.
- High Scalability:
- Established fermentation protocols allow for quick scale-up from research to industrial production.
- Flexibility:
- E. coli can be engineered to produce a wide range of proteins—from vaccine antigens to CRISPR enzymes.
Challenges:
- Regulatory Hurdles:
- As with any biopharmaceutical product, navigating regulatory approval is complex, particularly with forward-looking statements and the inherent uncertainties in clinical trials.
- Product Efficacy:
- The failure of certain vaccine candidates, as seen in the recent trial termination, underscores the challenges of translating preclinical success into effective therapies.
- Process Optimization:
- Achieving consistent yields and maintaining product quality across large-scale fermentations remain critical hurdles.
- Intellectual Property and Financial Risks:
- The significant investments made by companies such as Sanofi and J&J illustrate the high stakes involved in bringing these innovations to market.
Future Directions: Toward a New Era in Biomanufacturing
The landscape of E. coli-based biotechnology is evolving rapidly, driven by continuous research and development. Several trends and opportunities are poised to shape the future of this field:
Emerging Trends:
- Personalized Vaccines:
- Advances in genomics and bioinformatics may enable the design of vaccines tailored to individual genetic profiles, leveraging E. coli’s production capabilities.
- Integration with Digital Technologies:
- Automation, machine learning, and real-time process monitoring are expected to further optimize fermentation processes and quality control.
- Expanded CRISPR Applications:
- The development of “living diagnostics” and adaptive CRISPR systems could transform not only therapeutic gene editing but also environmental and clinical monitoring.
- Sustainable Production:
- Continued emphasis on green biotechnology may lead to innovations that reduce waste and energy consumption during large-scale fermentation.
Potential Clinical Impact:
- Enhanced Immunization Strategies:
- With needle-free transcutaneous immunization and improved antigen production, future vaccine delivery systems could become safer, more effective, and easier to administer.
- Broadening Therapeutic Modalities:
- The integration of synthetic gene circuits in E. coli production systems could enable the creation of multifunctional therapeutics that respond dynamically to patient needs.
- Cost Reduction and Accessibility:
- Optimized fermentation processes promise to lower production costs, making advanced therapies more accessible to a broader population.
Conclusion
The innovations in E. coli-based technologies are redefining the boundaries of vaccine development, enzyme production, and CRISPR enzyme manufacturing. Although challenges remain—ranging from regulatory uncertainties to process optimization—the potential benefits are immense. E. coli continues to serve as a robust platform for producing recombinant proteins, enabling cost-effective and scalable manufacturing solutions that have far-reaching implications in both clinical and industrial settings.
Summary of Key Innovations:
- E. coli DNA Vaccines:
- Despite setbacks in clinical trials, ongoing research and analysis pave the way for more effective vaccine candidates in the future.
- Enzyme Fermentation Insights:
- Studies on the impact of electrolysis products reveal novel methods to control bacterial cell division, with potential applications in industrial bioprocessing.
- Optimized Production Platforms:
- From small-scale expression to industrial-scale fermentation, E. coli remains a cornerstone in the production of DNA vaccine antigens and CRISPR enzymes.
- Transcutaneous Immunization:
- The needle-free patch approach shows promise in eliciting robust immune responses, offering a simpler and potentially more effective method for vaccine delivery.
- CRISPR Advancements:
- Leveraging E. coli’s innate sensor capabilities, innovative technologies like Record-seq are enhancing our ability to produce and optimize CRISPR/Cas enzymes, opening new avenues in gene editing and diagnostics.
As research and development continue to push the boundaries of what’s possible, the convergence of traditional microbiology with modern biomanufacturing techniques promises a new era in regenerative medicine and biopharmaceutical production. The journey is far from over, but each breakthrough brings us closer to more personalized, efficient, and accessible healthcare solutions.
References:
- Forward-looking statements and clinical updates on E. coli DNA vaccine studies –
- Trial termination and financial impact details from Sanofi and J&J –
- Electrolysis effects on E. coli cell division –
- E. coli-based antigen production and fermentation processes –
- Transcutaneous immunization strategy using E. coli-derived LT antigen –
- Record-seq technology and E. coli sensor applications in CRISPR enzyme production –
- Additional insights on CRISPR/Cas enzyme production –
The continued evolution of E. coli as a biotechnological platform highlights the interplay between innovative research and practical application. Whether through advanced vaccine production, optimized enzyme fermentation, or cutting-edge CRISPR diagnostics, E. coli-based technologies are poised to deliver transformative solutions that could reshape the future of healthcare and industrial biotechnology.
E. coli DNA Vaccines Enzyme Fermentation Recombinant Protein Production CRISPR Enzyme Production Transcutaneous Immunization Record-seq Technology Biomanufacturing Biotechnology Clinical Trials Vaccine Antigens Electrolysis Effects Regulatory Challenges Synthetic Biology Bioprocess Optimization