In recent years, bacterial biotechnology has emerged as a powerful driver of innovation across the pharmaceutical, industrial, and regenerative medicine landscapes. From pioneering synthetic approaches to protein synthesis to advanced CRISPR enzyme engineering and regenerative biomaterials, the bacterial platform is revolutionizing how we produce life-saving therapeutics and novel industrial enzymes. In this blog post, we delve into the cutting-edge strategies currently reshaping the field, examining the scientific breakthroughs and company collaborations that are propelling these advances forward.
Advances in Bacterial Biopolymer Synthesis
One of the most promising developments in bacterial biotechnology is the innovative work undertaken by Duke engineers on bacterial biopolymer synthesis. Traditionally, many biological therapeutics—such as antibodies, vaccines, and immune proteins—are produced in mammalian cell systems due to the sophisticated chemical machinery required for their assembly. However, the Duke team’s research introduces a synthetic approach designed to boost bacterial protein synthesis by creating “synthetic condensates.” These condensates act as engineered microenvironments that trap together the essential molecular components necessary for complex protein assembly.
This breakthrough holds promise for two significant industrial applications. First, by assembling the requisite machinery within a bacterial cell, it opens a route for bacteria to synthesize biologics that were previously the exclusive domain of mammalian systems. Second, these synthetic condensates can be designed to sequester the proteins being produced, thus protecting the host bacterium from potential toxic effects. Such protection is crucial for optimizing the production of antibiotics and other antimicrobial proteins, where intracellular toxicity has long posed a bottleneck in yield efficiency. This work not only provides a fresh perspective on bacterial protein production but also suggests a scalable route for producing high-value therapeutic proteins in an industrial setting.
Bacterial Cas Enzyme Fermentation for CRISPR Therapeutics
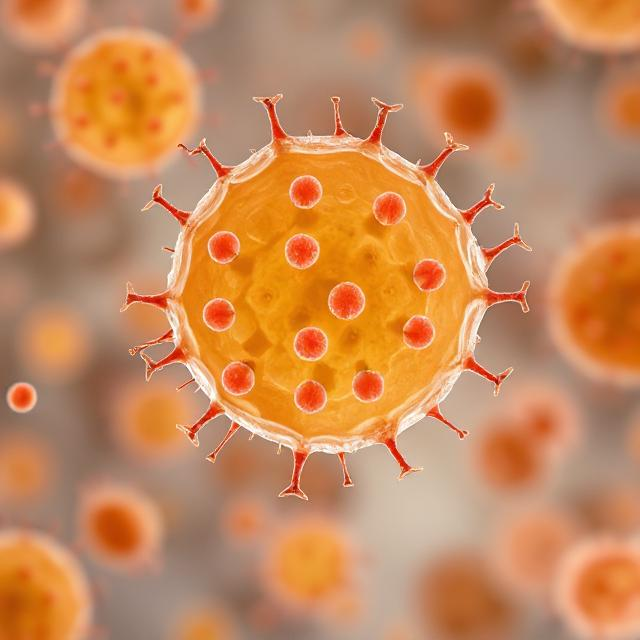
The evolution of CRISPR technologies has dramatically reshaped the landscape of genetic medicine. In particular, advancements in bacterial fermentation of Cas enzymes have had a profound impact on the development of next-generation CRISPR therapeutics. A notable example is the work spearheaded by Integrated DNA Technologies (IDT) on the Acidaminococcus sp. BV3L6 Cas12a enzyme. This system, originally published in 2015, attracted considerable attention due to its distinctive protospacer adjacent motif (PAM) of “TTTV,” which broadened the range of genomic targets compared to the well-known Cas9 enzyme with its “NGG” motif.
IDT’s breakthrough came with the development of Cas12a Ultra—a mutant that not only exhibits enhanced editing activity but also retains robust performance across a broader temperature range. This improvement positions Cas12a Ultra as a strong competitor to Cas9 and underscores the potential for bacterial fermentation processes to produce high-quality enzymes for genome editing applications. These advances have immediate implications in the clinical development space, where companies like CRISPR Therapeutics are leveraging bacterial enzyme fermentation to fuel their gene editing programs.
CRISPR Therapeutics has already demonstrated the clinical potential of CRISPR/Cas9 gene-edited therapies, particularly in treating hematologic diseases such as sickle cell disease and beta thalassemia. With partnerships involving industry leaders like Vertex Pharmaceuticals, CRISPR Therapeutics continues to push the envelope by integrating ultra-high performance enzymes into its clinical pipeline, thereby accelerating the transition from experimental therapy to transformative medicine.
Bacterial Cell-Free Protein Synthesis and Expression Systems
Another critical area of innovation involves bacterial cell-free protein synthesis systems. These systems offer a flexible platform for producing proteins without the constraints of cellular metabolism, thereby overcoming challenges associated with cell toxicity and metabolic burden. An exemplary case is the bacterial protein shuttling mechanism exemplified by the MacAB-TolC complex in gram-negative bacteria. This sophisticated protein assembly spans the cell’s inner and outer membranes and even the periplasm, effectively pumping out antibiotics and other small molecules. Such protein complexes not only allow bacteria to thrive in hostile environments by expelling harmful substances but also serve as a model for engineering cell-free systems that mimic these natural processes.
The intrinsic ability of bacteria to compartmentalize and secrete proteins has inspired researchers to develop novel strategies that use engineered protein expression systems to produce therapeutic proteins. For example, scientists have explored the design of synthetic condensates that mimic the natural sequestration mechanisms seen in bacterial cells. By channeling the target proteins into dedicated compartments, these systems reduce cytotoxicity and enhance production yields. This approach has significant implications for the industrial production of antimicrobial proteins and therapeutic enzymes, offering a pathway to more efficient and safer manufacturing processes.
Harnessing Bacterial Collagenase for Regenerative Medicine
In the realm of regenerative medicine, material innovations often hold the key to enabling breakthroughs in tissue engineering and cell culture technologies. Researchers at Tokyo Metropolitan University have recently taken inspiration from nature to develop a novel antibacterial material based on anodic porous alumina (APA). Mimicking the nanostructured surfaces found on cicada and dragonfly wings—known for their innate ability to resist bacterial colonization—the team engineered APA surfaces with precisely ordered arrays of alumina pillars.
These pillars are designed to disrupt bacterial cell membranes mechanically, thereby preventing bacterial proliferation without the need for chemical antibiotics. Importantly, these surfaces are not only highly antibacterial but also compatible with cell culture, making them a promising substrate for cultivating cells in regenerative medicine applications. The potential impact of this technology is far-reaching: by reducing reliance on antibiotics in cell cultures, APA surfaces may lower the risk of antibiotic resistance and enhance the safety of tissue-engineered products. Given that cell cultures are a critical step in the development of therapies for tissue and organ repair, this advancement may revolutionize the scale and safety of regenerative treatments.
Industrial Scale Bacterial Fermentation for Enzymes and Biofuels
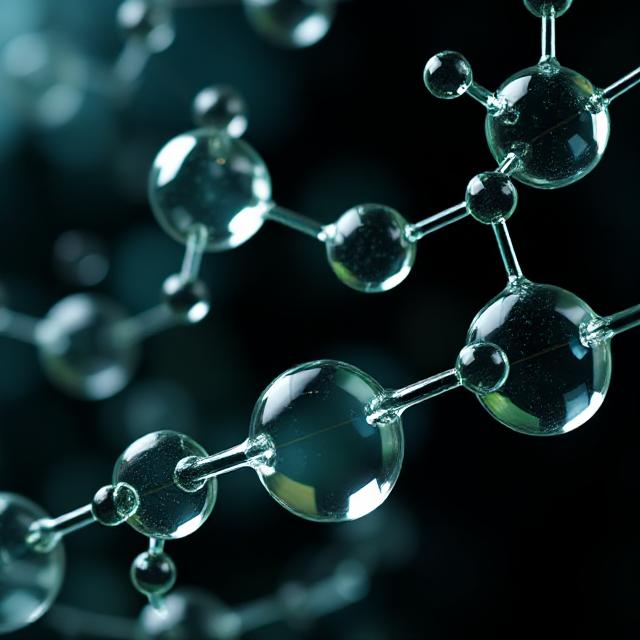
Beyond biomedical applications, bacterial fermentation continues to offer substantial benefits in industrial enzyme production and even biofuel generation. A recent study conducted at CNPEM’s pilot plant has demonstrated the potential for bacterial “super enzymes” to transform waste materials, such as sugarcane bagasse, into valuable biofuels. The research encompassed an extensive bioprospecting phase—identifying bacterial strains with inherent catalytic abilities—and culminated in the industrial-scale production of these enzymes.
The implications of this work are twofold. First, it highlights the feasibility of scaling up bacterial fermentation processes to meet industrial demands, reducing costs and increasing the accessibility of bio-based fuels. Second, the filing of a patent for the enzyme underscores the commercial potential of this technology, as licensing agreements may enable rapid adoption within the productive sector. As the industry moves towards more sustainable practices, the integration of bacterial fermentation systems for biofuel production represents a strategic pathway to converting agricultural waste into clean energy, thereby contributing to environmental sustainability and energy security.
Bacterial Protein Expression: From Phage Therapy to Antibiotic Resistance
Bacterial protein expression is a multifaceted field that has recently expanded into both therapeutic and diagnostic domains. One intriguing application involves the use of bacteriophages—the viruses that infect bacteria—to repurpose bacterial cells into tiny “protein pharmacies.” By reprogramming bacteria through phage infection, researchers have devised a strategy in which bacterial cells are transformed into efficient producers of targeted proteins. These proteins, once produced in high yields in the lower intestine, have the potential to modulate inflammatory responses and even address metabolic disorders such as obesity. This innovative approach leverages the natural replication cycle of phages to deliver therapeutic benefits directly at the site of action, thereby providing a novel avenue for addressing chronic inflammatory conditions.
At the same time, the intrinsic link between bacterial protein expression and antibiotic resistance has been a subject of intense study. In gram-negative bacteria, the MacAB-TolC multidrug efflux pump is a critical component in expelling antibiotics and virulence factors. An imbalance in the expression of this three-part protein complex can lead to enhanced resistance, as observed in recent research efforts. Understanding the molecular mechanisms behind this efflux system not only aids in the development of novel antimicrobial strategies but also provides insight into how bacteria evolve to survive in the presence of potent antibiotics. As antibiotic resistance continues to pose a global threat, advances in bacterial protein expression and efflux pump regulation remain at the forefront of microbiological research, driving both diagnostic and therapeutic innovation.
Exploring Bacterial Histone-like Proteins
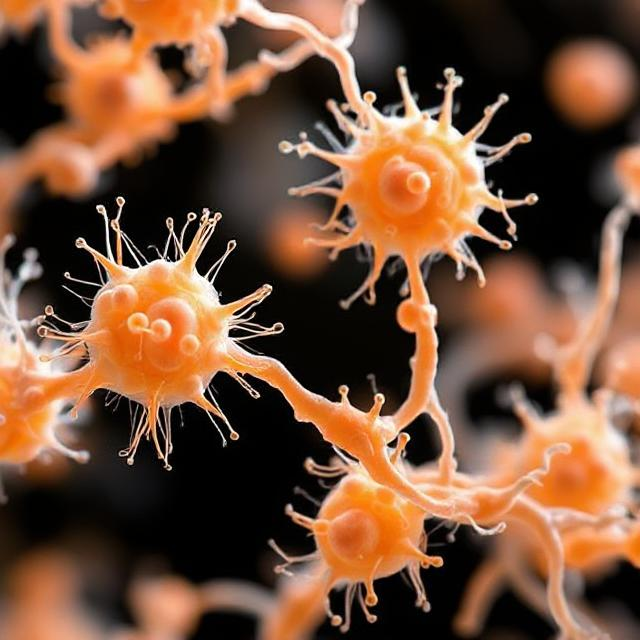
Recent investigations have also turned a spotlight on the discovery of histone-like proteins in bacteria—a finding that challenges traditional paradigms of DNA packaging and regulation. Unlike eukaryotic cells, bacteria were long thought to rely solely on simpler DNA-binding proteins for chromosomal organization. However, emerging research suggests that certain bacterial species possess histone-like proteins that play a role in organizing and protecting genomic DNA. One compelling study reported that these proteins might straighten the DNA strands, thereby preventing over-compression during cell division. This protective mechanism could be crucial not only for maintaining genomic stability but also for shielding bacterial DNA from bacteriophage attacks.
The potential dual functionality of these histone-like proteins—as both structural organizers and defensive shields—opens up intriguing avenues for further research. If these proteins can indeed make bacterial DNA “harder to get at,” they might be exploited in novel antimicrobial therapies aimed at sensitizing pathogenic bacteria to phage-mediated lysis or chemical treatments. Such a paradigm shift in our understanding of bacterial chromatin structure may eventually lead to the development of innovative approaches to combat antibiotic resistance and bacterial infections.
Synergistic Impacts and Future Directions
The diverse applications and technological innovations discussed above underscore the dynamic interplay between fundamental bacterial biology and its translational applications. The synthesis of biopolymers, fermentation of CRISPR enzymes, and advancements in cell-free protein synthesis are not isolated endeavors. Instead, they represent a continuum of research where each breakthrough informs and enhances the others.
For instance, the development of synthetic condensates for boosting bacterial protein synthesis is closely related to efforts in bacterial cell-free systems. Both approaches seek to overcome the intrinsic limitations of bacterial hosts by creating controlled environments that optimize protein production. Similarly, the work on bacterial Cas enzyme fermentation dovetails with larger trends in synthetic biology and genome engineering, where high-performance enzymes are critical for clinical success. Companies like IDT and CRISPR Therapeutics are leading the charge in this space, with strategic partnerships and aggressive R&D programs aimed at delivering next-generation therapeutics to patients worldwide.
Moreover, the integration of antibacterial surfaces inspired by natural nanostructures exemplifies how cross-disciplinary insights—from materials science to microbiology—can converge to solve pressing biomedical challenges. The collaboration between academic institutions, such as Tokyo Metropolitan University, and industrial partners is crucial for translating these laboratory innovations into commercially viable products that improve patient care.
Industrial applications also benefit from these scientific advances. Bacterial fermentation processes, whether for enzyme production or biofuel generation, are moving towards greater efficiency and sustainability. By harnessing naturally occurring bacterial systems and augmenting them with synthetic biology techniques, researchers are unlocking new possibilities for waste conversion and energy production—an essential step in meeting global environmental challenges.
Looking ahead, the future of bacterial biotechnology is bright. Emerging trends such as the integration of artificial intelligence for enzyme optimization, the use of microfluidic systems for rapid bioprocessing, and the exploration of novel bacterial species from extreme environments are set to further expand the boundaries of what is possible. These innovations promise not only to revolutionize therapeutic protein production but also to pave the way for entirely new classes of bio-based products.
Company Call Outs and Strategic Collaborations
Several industry leaders have played pivotal roles in these advancements, each contributing unique capabilities to the collective progress. Duke University’s pioneering research in synthetic condensate engineering has set the stage for reimagining bacterial protein synthesis, offering a blueprint for scalable production of complex therapeutics. Meanwhile, Integrated DNA Technologies (IDT) continues to innovate in the CRISPR enzyme space, with its Cas12a Ultra mutant providing enhanced genome editing performance that is critical for clinical applications.
CRISPR Therapeutics, a company that has evolved from a research-stage enterprise into a global leader in gene editing, exemplifies the potential of bacterial enzyme fermentation when combined with strategic corporate partnerships. Their work, in collaboration with Vertex Pharmaceuticals, highlights the synergy between academic research and industry expertise in accelerating the development of breakthrough therapies.
Tokyo Metropolitan University’s foray into designing antibacterial alumina surfaces further illustrates the transformative potential of combining nanotechnology with biological insights. This innovation, inspired by the natural antibacterial properties of insect wings, promises to reduce reliance on antibiotics in regenerative medicine—a significant step towards safer and more sustainable cell culture practices.
CNPEM’s pilot plant initiatives in Brazil showcase the industrial viability of bacterial fermentation for enzyme production and biofuel generation. Their efforts not only contribute to sustainable industrial practices but also underscore the global relevance of bacterial biotechnology in addressing both economic and environmental challenges.
Lastly, research from UCSF and other leading institutions continues to expand our understanding of bacterial protein expression mechanisms and antibiotic resistance. These studies provide the fundamental insights needed to develop next-generation antimicrobial strategies and to refine the use of bacteriophages in therapeutic applications.
Concluding Thoughts
The rapid pace of innovation in bacterial biotechnology is a testament to the ingenuity of researchers and the strategic vision of industry leaders. From synthetic condensates that enhance bacterial protein synthesis to high-performance CRISPR enzymes and next-generation antibacterial materials, these advances are reshaping our understanding of bacterial capabilities and opening up new avenues for therapeutic and industrial applications.
The interplay between basic scientific research and translational innovation is at the heart of these developments. Each breakthrough, whether in the lab or at the pilot plant, builds on a foundation of interdisciplinary collaboration and cutting-edge technology. As companies like IDT, CRISPR Therapeutics, and academic institutions like Duke University and Tokyo Metropolitan University continue to push the boundaries, we can expect to see an ever-expanding portfolio of bacterial-based solutions that address some of the most pressing challenges in medicine and industry.
Looking to the future, the integration of emerging technologies such as artificial intelligence, advanced microfluidics, and high-throughput screening will likely further accelerate progress in this field. As researchers uncover new bacterial species and harness novel biochemical pathways, the potential for innovative applications—ranging from improved therapeutics and diagnostic tools to sustainable industrial processes—will only grow.
In summary, the recent advances in bacterial biopolymer synthesis, CRISPR enzyme fermentation, cell-free protein synthesis, and antibacterial surface engineering illustrate a vibrant and rapidly evolving field. The collective impact of these innovations is profound, promising not only to transform how we produce biologics and industrial enzymes but also to pave the way for safer, more efficient, and environmentally sustainable technologies. With strategic collaborations, interdisciplinary research, and an unwavering commitment to innovation, bacterial biotechnology is poised to revolutionize the future of both medicine and industry.
References:
- Duke engineers’ synthetic approach for bacterial protein synthesis and its industrial potential –
- IDT’s development of the ultra-high performance Cas12a enzyme –
- CRISPR Therapeutics’ clinical advancements and strategic partnerships –
- Studies on the bacterial MacAB-TolC efflux pump and cell-free synthesis systems –
- Tokyo Metropolitan University’s research on antibacterial anodic porous alumina surfaces –
- Industrial-scale bacterial fermentation for enzyme production and biofuel generation –
- Research on bacterial protein expression via phage therapy and efflux mechanisms –
- Discovery of bacterial histone-like proteins and their implications –
Through these groundbreaking studies and collaborative efforts, the future of bacterial biotechnology looks set to yield innovative solutions that bridge the gap between fundamental research and real-world applications, offering hope for improved health outcomes and sustainable industrial practices worldwide.